Chapter 3: ý Memoryý |
Contents:
|
3.1 Structures and Models
 |
3.1 Structures and Models
Depending on the moment, our memory the register of our
experiences
‑ can be a source of frustration, of pain, of delight, or
of wonder. When we want to access it, often we cannot.
Sometimes, when we wish memories would fade, they will not.
At
unaccountable moments, sweet dreams may find their way into
our consciousness. Now and then, our sure recall of figure
or fact may allow us to act with uncommon confidence and
authority.
For as long as
we have thought about “human nature," that aspect
called "memory" has intrigued us. The scientific
study of memory is a recent matter, however, tracing back
only a little more than a century to the beginnings of
psychology as a systematic, experimental science.
The tradition
of memory research first begun by Ebbinghaus
dominated the study of memory for nearly a century
(1850-1909). In general, this tradition was based on the
following assumptions: (1) that words were the
primary mental units of language, (2) that when units were
used together they became linked and were chained into
larger units, (3) that complex behaviours and patterns of
thought were assembled from simple units, and (4) that the
mechanisms that produced learning and memory largely were
automatic.
Today,
however, our conception of what constitutes the valid study
of memory has been broadened considerably. Memory
theories based on rote memorization and extrapolation of
basic principles from simple to complex behaviour largely
have been supplanted by those that have attempted to
describe complex, meaningful cognitive processes more
directly. In the past three decades, especially, memory
theorists have made immense strides in describing the nature
of knowledge and in developing theories that permit
predictions about the nature of learning, memory, and
utilization of meaningful information.
|
3.2 Fundamental Distinctions in the Study of Memory
 |
3.2 Fundamental Distinctions in the Study of Memory
As cognitive
theorists began more and more to grapple with issues in the
learning and recall of meaningful materials, they quickly
faced questions about the nature of knowledge and how it is
stored in memory. Are there basic differences, for
instance, between "knowing" something and "knowing how to
do" something? Does personal experience lead to
different storage and retrieval than the more abstracted
general knowledge of, say, subject areas such as history and
chemistry? Is memory for language different than memory
for images? Are there differences between memory for events
just experienced and those experienced some time in the
past? Questions such as these have led to a number of
distinctions. Among the most useful and enduring have
been those between episodic and semantic memory, between
declarative and procedural knowledge, between language‑based
and imagery‑based systems in memory, and between short‑term
and long‑term memory.
Episodic versus
Semantic Memory

|
3.2.1 Episodic versus Semantic Memory
In proposing a
distinction between episodic and semantic memory,
Tulving argued for the utility of distinguishing
between the traces of personal experience, on one
hand, and general knowledge, on the other.
Specifically, episodic memory refers to storage
and retrieval of personally dated, autobiographical
experiences. Recall of childhood experiences,
recollection of the details of a conversation with a
friend, and remembering what you had for breakfast
all would fall within the realm of episodic memory.
The critical feature of episodic memory is the
existence of a "personal tag", and the basis for
retrieval is an association with a particular time
or place. Obviously, a great deal of what we must
recall in order to function effectively in our daily
lives is of an episodic nature.
Semantic memory,
in contrast, refers to memory of general concepts
and principles and their associations. Unlike
episodic memory, semantic memory is not linked to a
particular time and place. In our semantic, memory
is such information as the fact that lemons are
yellow and that computers contain chips. Semantic
memory contains the organized knowledge we have
about words and concepts and how they are
associated. For instance, a subject area such as
Egyptian literature or American history represents a
vast body of semantic information that we (as we
become more expert in the area) encode, organize,
and have available for retrieval. Recalling word
meanings, geographic locations, and chemical
formulas similarly requires searches of semantic
memory.
Although the
psychological validity of the episodic‑semantic
distinction has been criticized, it continues to be
useful in helping us think about the different types
of information we must remember. On one hand, the
episodic aspect of our memories must function well
enough for us to locate ourselves in time and space
and have a reasonably accurate record of our
experiences. At the same time, we have to have
available a general knowledge base in order to think
and reason effectively. Of course, the
episodic‑semantic distinction does not presuppose
two physically separate systems in the brain, but
rather is a conceptual distinction useful to
researchers and practitioners.
|
Declarative versus
Procedural Knowledge

Declarative memory

|
3.2.2 Declarative versus Procedural Knowledge
A second important
distinction in the study of memory is between
declarative and procedural knowledge. Declarative
knowledge is knowledge about facts and things,
knowledge that something is the case. In contrast,
procedural knowledge is knowledge about how to
perform certain cognitive activities, such as
reasoning, decision making, and problem solving.
Declarative memory
The memory
associated with cognitive skills not directly
attributable to muscular or glandular responses.
The complete memory may be acquired through a
single exposure, but practice is beneficial.
Declarative memory is required to recall factual
information, and it is sometimes called fact
memory. The ability to recognize a face, recall
a number, or recall any verbal or sensory
information requires declarative memory.
One important use
for the declarative‑procedural distinction is to
describe the kinds of learning students may achieve.
A novice student in a teacher education program, for
instance, may memorize principles of classroom
management (e.g., "Allow students to make value
judgments.") as declarative knowledge, but he may
have little or no notion of how these principles
actually would be used in effective teaching
(procedural knowledge).
Although it has not
been described with the terms declarative knowledge
and procedural knowledge, the declarative‑procedural
distinction has been implicit in the work of a
number of learning theorists‑for instance, in the
work of Benjamin Bloom and his associates. In
Bloom's analysis, for instance, a contrast was drawn
between lower levels of learning (i.e.,
knowledge, comprehension), in which facts, concepts,
and rules are learned and understood, and
"higher‑order" learning (i.e.,
application, analysis, synthesis, and evaluation),
in which knowledge is used as part of higher level
cognitive processes.
Of course, not all
procedural knowledge is "higher‑order" knowledge
based on more fundamental declarative knowledge.
Procedural knowledge can be quite simple and only
implicitly linked with declarative knowledge. A
young child, for instance, who remembers how to
unlatch the door, turn off a faucet, brush her
teeth, and open a book, is showing her recall of
procedural knowledge.
Also, procedural
knowledge often is "automated" we often begin
"doing" without any apparent conscious attention
to what we are doing or why we are doing it. In a
lecture class at a university, for example, most
students will enter the class, find a seat, take out
a notebook, and begin taking notes with little or no
conscious attention to the task. Similarly, as we
read, decoding words and comprehending the meaning
of what we are reading ordinarily occurs quite
automatically. Sometimes, however, our searches of
declarative knowledge come at least partially under
conscious control. ("Who is the author of Hamlet?")
In most learning,
of course, there is interplay between declarative
and procedural knowledge. A concert pianist
learning a new song by Domenico Scarlatti,
for instance, may search her memory for declarative
knowledge about that composer's preferred method of
executing certain embellishments such as the
appoggiatura, mordent, and trill‑declarative
knowledge that will be utilized in the development
of procedural knowledge. Conversely, procedural
knowledge has undeniable impact on declarative
knowledge. Like most experts, our pianist has
procedural knowledge about how she best recalls
information about composers and their works and will
search her declarative knowledge accordingly. Yet
another cluster of procedural knowledge‑her skills
in performing‑enhances and gives substance to the
declarative knowledge she possesses (e.g.,
"Scarlatti intended for the mordents to be played
according to the basic tempo of the passage. That
would mean that they should be thirty‑second notes
here.")
In most school
learning, similarly, there will be goals
for the acquisition of both declarative and
procedural knowledge. One important goal of
education is the development of relatively large,
stable, and interrelated sets of declarative
knowledge. As educators, we expect students will
be "knowledgeable". At the same time, however, we
place a considerable premium on knowing "how to."
For the practitioner, usable knowledge is critical.
Especially in applied programs such as journalism,
architecture, teaching, management business, and
medicine, procedural knowledge is an important
outcome of the educational process.
|
Verbal and Imaginal
Representation in Memory

|
3.2.3 Verbal and Imaginal Representation in Memory
"A picture is worth
a thousand words."
Although the validity of this aphorism may be
debatable, there is little doubt that we humans have
extraordinary capabilities for remembering
information about visual events. There is little
doubt that pictorial information can be represented
in our memories quite well. Certainly, our
subjective experiences would tell its so. Most of us
easily can conjure up an image of a book, a soaring
bird, a train wreck, or a walk in the woods.
One of the main
contributions of cognitive
psychology has been a revitalization of
interest in the study of mental imagery. Once
largely banished from experimental psychology as
subjective, mentalistic, and therefore unscientific,
imagery has become a significant feature of the work
of a number of cognitive psychologists.
One such
psychologist, Alan Paivio, has proposed
that information can be represented in two
fundamentally distinct systems, one
suited to verbal information and the other to
images. The verbal coding system is adapted for
linguistically based information and emphasizes
verbal associations. According to Paivio, words,
sentences, the content of conversations, and stories
are coded within this system. In contrast,
nonverbal information is stored within the imaginal
coding system. Pictures, sensations, and sounds are
coded here.
Paivio's theory
has been called a dual coding theory, in that
incoming information can be coded within one or both
of the systems. Although the systems are separate,
they are strongly interconnected in their impact on
the recall ability of information. To the extent
that information can be coded into both systems,
memory will be enhanced, whereas information coded
only in the verbal system or imaginal system will be
less well recalled. In Paivio's view, the
verbal and nonverbal codes basically are
functionally independent and "contribute additively
to memory performance". Paivio also
hypothesizes that nonverbal components of memory
traces generally are stronger than verbal memories.
Much of Paivio's
early work
was devoted to demonstrating the effects of the
abstractness of materials on its memorability and
relating these results to dual coding theory.
For instance, some words (bird, star, ball, and
desk) have concrete referents and presumably are
highly imaginable. Thus, when presented with such
words, both the verbal (e.g., the linguistic
representation of the word bird, its pronunciation,
its meaning) and the imaginal (an image of a bird
soaring) representations are activated
simultaneously. Other words, however, are more
abstract and far less readily imaginable (e.g.,
aspect, value, unable). These words, although they
activate the verbal coding system, are hypothesized
to activate the nonverbal system only minimally. In
Paivio's view, memory for abstract materials should
be poorer since such materials are represented only
within a single system. Pictures, since they tend to
be automatically labeled, should be more memorable
than words because, although pictures are
automatically labeled (and hence dual‑coded), words,
even concrete ones, are not necessarily
automatically imaged.
In a large number
of studies,
Paivio and his associates have demonstrated
the beneficial effects of imagery on learning and
memory, consistent with his predictions. Words rated
high in imagery have been shown to be better
remembered in free recall, in serial learning (where
a series of words must be recalled in order), and in
paired‑associate learning (in which the "associate"
of a word must be recalled when the word is
presented). Similarly, instructions to subjects to
"form images" also have been shown to enhance
memory. |
|
3.3 Mental Rotation
 |
3.3 Mental Rotation
An
intriguing set of studies carried out by Roger Shepard
and his associates has provided additional information about
the nature of mental images, their distinctiveness
from verbal information, and the role they play in
cognition. In an early study, Shepard had subjects
think about such questions as the number of windows in their
house. He noted that the time required to produce an
answer increased with the number of windows counted,
consistent with the idea that individuals actually were
mentally manipulating some sort of image. Further, subjects
described themselves as taking a "mental tour" of
their house in order to respond to this question. At least
subjectively, there was a strong impression of mentally
picturing - looking at or scanning - images.
In a
later series of studies, Shepard and his co‑workers showed
that mental images generated by persons underlie a number
of cognitive operations. In one set of studies, for
example, persons were asked to judge whether
three‑dimensional objects presented in different
orientations were identical; see Figure 3.1. The fascinating
result was that the time required to make the judgments
increased linearly with the extent of rotation required.
That is, it appeared that persons were mentally rotating the
objects in order to make the comparison; the greater the
rotation, the longer it took to make a judgment.
More
recently, Stephen Kosslyn and his colleagues have
demonstrated other interesting effects. For example, in one
study, persons were asked to memorize a map of an island on
which such objects as a tree, rock, or hut were depicted at
varying locations; see Figure 3.2. After the map was
committed to memory, they were asked to focus on a named
object on the map. They then were given the name of a second
object and told to locate it by imagining a black speck
moving in a straight line from the first object to the
second. Objects were, of course, varying distances from one
another on the map. If the mental image is being scanned, as
Kosslyn hypothesized, then time required to move from
one object to the next should vary directly with the
distance on the image. In fact, this was what Kosslyn
and his associates found. "Distant" objects took
longer to reach than "near" objects, demonstrating that
images, like pictures, contain information about the spatial
relations among objects.
Figure 3.1: Pairs of
patterns with different orientation.
These
pairs of figures are similar to those used by Shepard and
XletzIer (1971) in their study of the mental rotation of
three‑dimensional objects.
Figure 3.2: An island map.
Using persons' ability to
form images of different sizes (e.g., a large rabbit versus
a very tiny rabbit) and at different locations (e.g., nearby
versus far away), Kosslyn also has shown that when
persons are asked to verify certain features of mental
images (e.g., "Do rabbits have whiskers?"), details
of "small" images (e.g., a small rabbit) take longer
to verify than those of "large" images (a large rabbit).
According to Kosslyn, such evidence points to the conclusion
that images have a "grain" or resolution. Thus,
portions of images visualized as subjectively smaller
actually make details harder to discern.
|
3.4 Short‑Term versus Long‑Term Memory
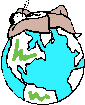 |
Short‑Term versus Long‑Term Memory
Beginning in the late 1950s and increasing rapidly
thereafter, the research journals in learning and memory
began to be flooded with research on a new topic. What
was being studied and reported on was not a new phenomenon,
but a new dimension of the already well studied area of
human memory. The new dimension being investigated was
the nature of memory over very short intervals‑seconds or
minutes. The name given to this phenomenon was
short‑term memory, or simply STM.
Memory
theorists long had proposed that there may not be one,
but two, mechanisms for memory storage. What they
suggested was that one type of storage mechanism is
available for events recently experienced. This
mechanism is the realm of STM. Another type of storage
system seems to exist, however, for traces of experiences
developed over longer periods through repetition, habit, and
study. This aspect of memory is called long‑term memory,
or LTM.
Several differences between STM and LTM
were hypothesized. First, it was contended that STM
involves "activity" traces in contrast to LTM's "structural"
traces. That is, STM is dependent on ongoing electrochemical
brain activity; in contrast, LTM is based on relatively
permanent changes in brain cell structure. Another,
related contention was that STM decays autonomously,
whenever attention is diverted from what is to be
remembered. LTM, however, is based on irreversible,
non-decaying traces. Third, obvious differences in
capacity between STM and LTM were noted. Whereas STM has
relatively fixed limits, LTM was judged to have apparently
unlimited capacity.
These distinctions match well with our own introspective
assessment of our memory capabilities.
For instance, when we encounter new information, we
generally need to continue to pay attention to it and
rehearse it in order to "keep it in mind."
Remembering a phone number we have just looked up or the
names of several new acquaintances, for most of us,
requires some attention and repetition. Especially on
first encounter, our memory for such information can be
exceedingly fragile‑even a brief interruption or distraction
may cause us to loose the thought entirely.
Once information has been well learned and committed to
memory, however, rehearsal and repetition seem much less
critical. We
easily can state our uncles' names, recall the names of two
large cities on the Red Sea, or give three examples of large
hairy animals without having to rehearse any of this
information‑despite the fact that we may not have thought of
these topics for months or even years!
In
more recent models of memory, however, the importance of
the STM‑LTM distinction has diminished. Although memory
theorists have continued to pay attention to the differences
between STM and LTM, most models of memory have shifted from
storage to a "processing" emphasis. This processing
emphasis is retained in most current models. Rather than
being conceived of as a "place" where information is held
for brief periods, the concept of STM has been broadened so
that it reflects the many different ways in which we deal
with information. The STM now more and more reflects the
concept of "working memory"‑that part of our cognitive
systems we would refer to as our consciousness. For example,
J.R. Anderson's ACT model incorporates a
"working memory" and a long‑term memory." These two are
not emphasized as "separate places," however, but rather as
being closely interrelated. The current contents of
consciousness set up a pattern of activation in LTM; this
activation of LTM, in turn, may "reverberate" back into
working memory.
|
3.5 Concepts

|
3.5 Concepts
One
of the major ways in which we deal with the bewildering
array of information in the world is to form categories.
Our language mirrors these categories‑the words grandfather,
data, bird, psychology, red, dog, and man each represent a
category meaningful to most of us. Concepts are the
mental structures by which we represent these categories.
Particular objects or events are grouped together based
on perceived similarities; those that "fit" the category are
examples or instances of the concept; those that do not are
non examples. The similar features across examples of a
concept (e.g., all oceans contain water and are large)
are called attributes; features essential to defining the
concept are called defining attributes. Learning
concepts involves discovering the defining attributes along
with discovering the rule or rules that relate the
attributes to one another.
The
work of Bruner and others has shown that individuals
typically solve concept identification problems by trying to
discover the rules relating the concept attributes. In
general, concepts that have more difficult rules are more
difficult to learn. The simplest rules involve
affirmation (e.g., any green object) and negation
(e.g., any object that is not green), which apply if there
is only one attribute being considered. Most concepts,
however, involve more than one relevant attribute and hence
more complex rules. Among the most common are conjunctive
rules, in which two or more attributes must be present
(e.g., any triangle that is green), and disjunctive rules,
in which an object is an example of a concept if it has one
or the other attribute (e.g., either a triangle OR a green
object).
In recent years, Bourne's work has represented the
clearest statement of rule‑governed conceptual structure. In
his view, concepts are differentiated from one another on
the basis of rules such as the above. These rules provide
the means for classifying new instances as either linked to
a concept or not. According to Bourne, membership in a
conceptual class (e.g., grandfathers, data, and birds) is
determined by applying a set of rules. These rules can be
learned either through instruction or through experience
with instances that either are members of the class
(positive instances) or are not (negative instances). Thus,
one learns to classify a set of animals as birds or nor
birds on the basis of instruction or experience that leads
to acquiring rules for combining characteristic attributes
of birds (e.g., wings, bills, feathers). Instruction,
according to Bourne, should involve presentation of
both positive and negative instances (e.g., for birds,
pigeons versus bats) so that critical attributes clearly can
be linked to the concept. Presumably, use of these rules
unambiguously classifies a new instance as either a bird or
nonbird. Note, however, that this classification is a very
simple one‑a new instance either is a bird or is something
else, a nonbird!
Although a rule‑based
conceptual system works to organize information for many
concepts, it is inadequate for others. Most natural or
"real‑world" concepts are more "fuzzy" and differ
qualitatively from those studied in the laboratory. For
instance, consider the concept furniture. Our past
experience would let all of us quickly agree that furniture
includes tables, chairs, sofas, and floor lamps.
Furthermore, we can describe many rules that differentiate
articles of furniture from other objects. But some of our
attempts at rule formation quickly run into trouble.
Presence of legs? But what about some floor lamps? A
seating surface? But what about tables or a desk? Is rug
furniture? Some would say that it is, but would wish to
include a Qualifying statement or "hedge"‑it is like
furniture, but not exactly like it. What is the set of rules
that unambiguously determine which objects are members of
the concept class furniture? Logical efforts to determine
such sets of rules mostly have been unsuccessful, especially
with ambiguous examples such as Rug. Rosch and Mervi,
dissatisfied both with the artificiality of laboratory work
on concept formation and with the difficulties of
classifying concepts with rule‑governed approaches, proposed
an alternative view based on‑degree of family resemblance"
to a highly typical instance of the concept, a prototype.
|
3.6 Analogy and Metaphor Comprehension
 |
3.6 Analogy and
Metaphor Comprehension
Analogy is to human
reasoning as bricklaying is too human building.
In conjunction with categorization, it is one of the
principal means by which knowledge about the world is
acquired and structured. As a result, considerable effort
has been put into the understanding of analogy by a variety
of disciplines. Verbal analogies have been the
target of a considerable amount of that attention, and such
research has begun to be integrated with research on
metaphor comprehension. The requirement for integration
arises because it is known that some analogies can be
metaphorical, and because metaphors can often be
regarded as analogies. One view of metaphor is that it
constitutes a mapping of the elements of one set on to
another; and that it is the use of a given relation in a
group of things to facilitate the discrimination of an
analogous relation in another group. Simple, or sentential,
metaphors are assumed to be represented by simple,
proportional analogies (A: B: C: D), whereas extended
metaphors, or models, require more complex analogical
representation. There are many accounts of the nature of the
relationship between analogy and metaphor, to which the
interested reader is directed.
Analogical thinking,
in the general propositional view, is a means of
recording similarities between elements that already
exist in the knowledge base, and whose properties are
static; subject to a set of constraints. There is a
similarity between this and a view of metaphor in which
comprehension is seen to proceed by (a) a retrieval of
sets of semantic features that are propositional in nature,
and (b) a selection from these features of an appropriate
‘common ground’ for the metaphor. In terms of Miller,
the propositional view of metaphor is more akin to the
construction of semantic models than it is of memory images.
From the point of view of cognitive psychology, we come
to know things by gathering, processing, and storing
information. This is accomplished through sensation
and perception, learning and memory, and thinking.
Thinking involves mentally acting upon the information that
senses, perceives, learns, and stores.
What do
you mean when you say that we mentally act on information?
Suppose you are Dave Bowman and,
upset by the death of your fellow astronauts, you cloister
yourself and a colleague inside a cubicle, away (so you
assume) from the discerning ear of Hal.
(Unfortunately, best known to you, Hal also has a discerning
eye that is adroit at
up‑reading.)
As you discuss the
astronauts'
deaths and other computer‑related problems,
you mentally picture Hal's computer console and the
countless wires,
computer chips, and other electronic hardware comprising
Hal. You recall strange events and snatches of
conversations you've heard in the past few days and start
drawing connections between them. You trace the problems to
Hal. You discuss ways to remedy the problems and decide on
one: disconnect the source of the problems.
What have you been doing?
You have been thinking. You have been using information that
was previously gathered and stored and have been mentally
acting on it by forming ideas, reasoning, solving problems,
drawing conclusions, making decisions, expressing your
thoughts, and comprehending the thoughts of others. Thinking
involves a variety of mental processes and operations. The
ones we will examine here are mental imagery, problem
solving,
and
creativity. But before we get to these topics, we must
address the large issue of how we think. By what means do we
encode incoming information so that we can think about it?
|
3.7 How Do We Think? Pictures and Words
 |
3.7 How Do We
Think? Pictures and Words
Think about these two very different sentences:
|
1. The bulbous blue hippopotamus, reeking
from the odour of stale fishy brine, waddled into
the room and plopped onto the floor with a
self‑satisfied grin spreading over its face.
|
|
2. Our nation was conceived in a spirit of
unity for all time, freedom from persecution,
equality for the populace, and justice unequivocable.
|
After
reading the first sentence, could you just "see" the hippo
walking through the room? Were you almost disgusted at the
fish
odour? Could you
"feel" the vibrations when the hippo plopped to the floor?
Flow about the second sentence, could you "see" unity?
Freedom? Justice? How do we represent information in our
minds? Do we think in pictures as the sentence about the
blue hippo illustrates, the answer seems to be yes. But most
of us probably didn't call to mind any mental pictures when
we read about the abstract concepts of justice and equality,
yet we still understood what was being said.
There is some controversy over how information is
represented in our minds.
Some experts believe we encode information about real
objects and events into mental representations of those
objects and events.
When
we think, we mentally manipulate these
mental images.
Others believe that we encode information in terms of verbal
descriptions called propositions and that mental images are
sometimes added to the propositions after they are retrieved
from memory.
A proposition is defined as the smallest unit of knowledge
that can be validated as true or false.
Even though propositions are really abstract cognitive
events, most propositional theories depict them as short
sentences, such as "Clinton is president." John
Anderson has proposed a theory called adaptive control
of thought (ACT) based on propositions. Anderson envisions
propositions at the nodes of a net with all strands of the
net leading to propositions. In this way, all thought
processes are made up of propositions or combinations of
propositions. Allan Paivio has combined
mental images and verbal images (propositions) into a theory
of cognitive processing known as the dual-coding hypothesis.
3.7.1 The
Dual‑Coding Hypothesis
 |
3.7.1 The
Dual‑Coding Hypothesis
According to the dual‑coding hypothesis,
information is encoded by means of both an imagery
system and a verbal system, each working
independently. We use the imagery system for
processing real, concrete items and pictures, such
as blue hippos and a painting of the Mona Lisa.
We use the verbal system for more abstract items,
such as spoken or written words and concepts such as
liberty. So the imagery system is specialized for
processing information about nonverbal objects and
events, whereas the verbal system is specialized for
processing linguistic information and generating
speech. |
|
|
|
|